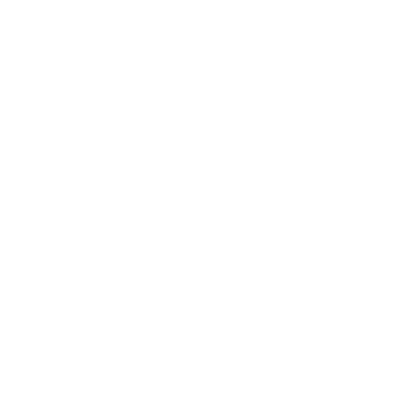
MOLECULE

- Free Radical scavenger
- Physical Quencher of excited states
- Free Iron and Copper chelator
- MMP inhibitor
Regimen Lab Skincare Encyclopedia
Acetyl Zingerone
V 1.0 last edited 25Sep2020
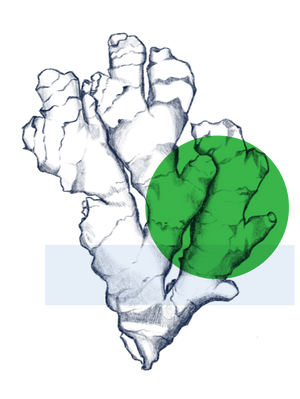
Regimen's Take
Acetyl Zingerone is an up-and-coming ingredient with many published studies investigating its mechanism of action and its effect on oxidative stress. It shows excellent antioxidant, quenching and scavenging property in vitro and the fact that it can prevent CPD formation is quite impressive. It offers protection against Blue light and Pollution-induced ROS formation as well. Several in-vitro, mouse in-vivo and genomic studies have been performed to elucidate its detailed biochemical mechanism of action and docking on target proteins. How well this translates clinically is still up for debate. Since it is relatively new, there is only one clinical trial involving its efficacy.
TLDR
- Acetyl Zingerone is a phenolic alkanone that is derived from ginger (Zingiber officinale).
- It is an all-around active that acts as a potent antioxidant, anti-inflammatory, chelator of transition metals, protector of ECM, scavenger of free radicals, and quencher of high energy DNA-damaging molecules.
- AZ’s main differentiator is its ability to reduce Dark DNA damage (dark CPD) hours after sun exposure.
What is Acetyl Zingerone?
Zingerone is one of the antioxidant components of ginger that has been studied for various properties in both mice and humans. Ancient experiments on eastern medicine noted that roasted ginger is better in alleviating various symptoms. Interestingly, it was found that fresh ginger contains high amounts of a compound called Gingerone, which converts to Zingerone on drying or cooking. Gingerone and Zingerone both share the same structure with Curcumin (the active ingredient in Turmeric), giving them similar pharmacologic effects. Acetyl Zingerone has an added acetyl group (methyl group single-bonded to a carbonyl), which confers additional scavenging, chelating, and stabilizing potential to Zingerone. AZ is intentionally engineered to neutralize key Radical Oxygen species (ROS), Non-radical Oxygen species (singlet oxygen), and strong nucleophiles (peroxynitrite) that are induced by UVR. It is reported to have the following properties:
How does it work?
Antioxidant
When our skin gets exposed to UV, harmful Radical Oxygen Species (ROS) gets produced. These radicals can damage proteins, DNA, and other cellular structures in the skin. This ROS increase leads to the increase of the MMP-1 enzyme, which degrades collagen, leading to the appearance of wrinkles.[5]
UVA and or UVB radiation induces the release of IL-1, IL-10, TGF-B1, and TNF-a, which are molecules that promote inflammation. These cause the production of Nitric Oxide (NO•), which is another harmful free radical. NO• radicals are produced from Nitric Oxide Synthase (NOS) enzymes, and there are three types found in the skin:[6][7][8][9]
- Keratinocytes express neuronal isoform of NO• synthase (nNOS)
- Fibroblasts and other cell types in the skin express endothelial isoform (eNOS)
- Inducible form of NO• synthase (iNOS) that activate under UV and other conditions
UVB alone, without the presence of the previously mentioned pro-inflammatory cytokines, can also induce iNOS. NO•, as a result, is correlated with inflammatory skin conditions as well as erythema, edema, and stimulation of melanogenesis.
Acetyl Zingerone is shown to be 250% more effective in reducing iNOS activity than Zingerone, which is well studied antioxidant. UV-induced DNA damage was assessed through gel electrophoresis, and the results show that AZ has protective properties through physical QPES (Quenchers of Photoexcited States) mechanism. AZ also regulates genes responsible for reactive oxygen species metabolism (PMAIP1, ARG2).[10]
The mitochondria are well known to be the cell’s powerhouse as it supplies the energy needed for various processes. Hence, the Mitochondrial DNA (mtDNA) codes for many critical biochemical processes in the skin. Although it acquires the same DNA damage as nuclear DNA, it does not possess the full range of DNA repair mechanisms that operate in the nucleus. Some researchers proposed the mitochondrial clock of aging, which is based upon the progressive accumulation of this oxidative damage, especially the ones arising from ROS.[11] In short, they argue that the reason we age is that our mitochondria accumulate damage from ROS without having the ability to repair itself, unlike nuclear DNA.
More recently, it was found that specific deletion of the 4977 base pair severely alters energy production and cellular function in mitochondria.[12] For this reason, physical Quencher of Photo-excited States (QPES) compounds have the potential to reduce skin photocarcinogenesis and photoaging.
To assess whether AZ has any mitochondrial protective ability, an MTT assay was performed. Succinate dehydrogenase is one of the enzymes in the electron transport chain that produces energy in mitochondria. Hence, it was used assayed to monitor mtDNA caused by UV. The results show that even at low concentration AZ improved cell metabolism and almost completely reversed ATP-damage and plasma membrane disruption. AZ has a marked and significant effect in preventing and reversing UV radiation-induced mtDNA damage.[10:1]
As previously discussed, ROS are damaging radicals that wreak havoc in the skin and preventing, scavenging, and quenching them is beneficial to the skin. AZ’s ability to neutralize ROS was assessed using keratinocytes, and the results suggest that AZ significantly reduced ROS formation in a dose-dependent manner by -35% and -46% for low dose and high dose, respectively. The same study assessed AZ’s scavenging activity on common ROS compared to known antioxidants such as alpha-tocopherol and Resveratrol, and found AZ was superior to a-tocopherol and Resveratrol. AZ was 2.72 times more active than a-tocopherol in scavenging singlet oxygen, 17.7 times greater in scavenging peroxyl radical, 103 times greater for hydroxyl radical, and 127 times greater in scavenging peroxynitrite.[2:2]
Compound |
Peroxyl |
Hydroxyl |
Superoxide |
Singlet Oxygen |
Peroxynitrite |
Lipid Peroxide |
Acetyl ZIngerone |
25,325 |
9,723 |
- |
7,180 |
1,139 |
0.48 |
α-Tocopherol |
1,432 |
94 |
- |
2,636 |
9 |
36.3 |
Resveratrol |
68.9 |
20.7 |
5.3 |
45.6 |
2.7 |
0.43 |
Results expressed as µm Trolox equivalent/g; For Lipid Peroxide, IC50 in µg/ml. AZ reduces Superoxide by inhibiting NADPH oxidase.
There are two types of quenchers (molecules that relaxes the excited free radicals) for electronically excited singlet oxygen (1O2, one of the major ROS): chemical quenchers and physical quenchers.[13] Chemical quenchers like ascorbic acid (Vit C) react chemically with 1O2 while being sacrificed in the process.[14] In contrast, physical quenchers like Carotenoids[15][16][17] and Acetyl Zingerone relaxes 1O2 back to its 3O2 ground state through an energy transfer process without being chemically degraded.
Some antioxidants are known to possess physical and chemical quenching with most of their scavenging activity involving chemical quenching. Most physical quenchers are usually electron-rich compounds such as amines, phenols, and methoxybenzene. The generally accepted mechanism for physical quenching is through an exciplex (an excited complex of an electron donor and an electron acceptor) between the organic compound and 1O2. This process involves varying degrees of charge transfer character with eventual decay by intersystem crossing to relax the complex into oxygen (3O2) and the organic compound in their ground states.[18] This means that AZ and 1O2 form an AZ-1O2 complex where several energy transfers from the excited 1O2 to AZ happens. In this way, both the excited 1O2 and AZ return to their unexcited ground state without being degraded. This mechanism explains why AZ works better than tocopherol and at quenching singlet oxygen, as it doesn’t degrade after reacting with singlet oxygen. These findings were supported by the HPLC detection of more stable AZ, compared to alpha-Tocopherol while reacting with singlet oxygen.[1:2]
Most antioxidants show good scavenging results in chemical assays; however, some have significant absorbance in the UVA (320 -400 nm) and UVB (290 – 320 nm) regions. They absorb the energy from UVR and either die during the process or become pro-oxidants that increase, rather than decrease, ROS formation.[19] There are several considerations when picking antioxidants because not all of them fulfill the Regimen’s Antioxidant Criteria. Hence, it is essential to have multiple antioxidants to cover the shortcomings of others. AZ has negligible absorbance in the UVA and UVB region where it mainly absorbs at 280 nm, so it doesn’t turn into a pro-oxidant upon UV exposure.[1:3]
One of AZ’s key features is its ability to neutralize peroxynitrite (ONOO- is a byproduct of ROS which is capable of producing more DNA damage) in high efficiencies. ONOO- reacts as an oxidant and nucleophile[20] by reacting with enolizable carbonyls such as 3-methyl-2,4-pentanedione through either one-electron oxidation or nucleophilic addition to the carbonyl group.[21] Since AZ was engineered to have an enolizable carbonyl group, it functions as a ONOO- neutralizer. Chemical assays show that it neutralized ONOO- 127 times more efficient as alpha-tocopherol with 1139 um Trolox equivalent per gram compared to 9 um Trolox equivalent per gram of tocopherol and 2.7 um Trolox equivalent for Resveratrol.[1:4]
Analyzing AZ’s molecular structure and comparing it with the known structure and docking studies done on Zingerone, we see that Z and Curcumin both serve as a scaffold for AZ in which:
- Phenol rings with hydroxyl groups as a proton donor antioxidant
- Methoxy group as a single oxygen quencher
- 2-butanone branch chain as a peroxynitrite scavenger
AZ was engineered with a 2,4-dione branching chain added to Z, which confers hydrogen bond chelator functionality as well as improved pharmacological properties, photostability, scavenging efficacy, and quencher of photoexcited states.[1:5]
Chelator
Exposure to UV causes the immediate release of iron in the skin from ferritin, an iron-storage protein. The released iron then reacts with H2O2 to form OH• radical. Ascorbic acid is also known to reduce ferric Fe3+ to ferrous Fe2+ ions (and Cu2+ to Cu+), which can reduce hydrogen peroxide (H2O2) to hydroxyl radicals OH•.[22]
AZ was compared to common chelators of transition metals using a decrease in fluorescence of fluorescein, indicating the presence of OH•. The results showed that AZ was far superior to EDTA and more effective than Deferiprone, which is one of the most potent iron-chelating agents known. Chelating iron is mainly beneficial in inhibiting OH• production from the Fenton Reaction. Therefore the presence of AZ would lessen the likelihood of antioxidants turning into pro-oxidants.
Lipid peroxidation
After skin exposure to UV, ROS generation triggers the oxidative degradation of glycerophospholipids, sphingolipids (e.g. ceramides), unsaturated fatty acids, and cholesterol.[23] This means that the intercellular lipids of the moisture barrier become damaged upon UV exposure. The byproducts of lipid peroxidation are known to be toxic, mutagenic, or even carcinogenic. Lipid peroxidation can be prevented by using certain antioxidants capable of inhibiting the process.
Zingerone was previously shown to inhibit lipid peroxidation in vitro and in rat in vivo studies.[24][25][26][27][28] Acetyl Zingerone was hypothesized to have the same effect on lipid peroxidation as Z. AZ was compared with a-tocopherol and Resveratrol in inhibiting lipid peroxide, and results showed that AZ has an IC50 of 0.48ug/ml with squalane as a substrate. In contrast, a-tocopherol has an IC50 of 36.3, and Resveratrol of 0.43, indicating that AZ was far superior to a-tocopherol, while being comparable to Resveratrol’s ability to inhibit lipid peroxidation.[1:6]
DNA damage protection
DNA damage from UV exposure is known to cause significant harm to the skin. Visible signs of DNA damage include sunspots, sunburn, wrinkles, and cancer DNA structure is akin to a zipper, and UV exposure causes kinks to form that prevents the “zipper” from functioning.
One of the most common UV-induced kinks is Cyclobutane Pyrimidine Dimers (CPDs). CPDs constitute approximately 80% of all lesions induced or formed by UV radiation exposure. These are covalent linkages between consecutive bases along the DNA chain in the vicinity of their carbon-carbon double bond.[29] Like kinks in a zipper, they can cause errors in the DNA during replication and transcription.
CPDs have been identified as the leading cause of melanoma and non-melanoma skin cancers.[30] They are also linked to photoaging[31][32], production of immunomodulatory cytokine TNF-a[33], and onset of erythema (redness) after sun exposure.[34] The past understanding of DNA damage was that it only happens during active UV exposure when DNA directly absorbs UV. It was thought that after DNA absorbs UV, it forms electronically excited state species that subsequently undergo photochemical transformation to form light-CPDs (CPDs forms in the presence of UV “light”).[30:1] However, newer studies show that DNA damage continued to increase by 50% or more after irradiation ends, with or without light and independent of additional UVR photons.[35] A more recent study showed that these dark-CPDs (DNA damage) occurred both in vitro and in vivo in human skin within the first two hours of exposure to UVA/visible radiation.[36][37]
The mechanism for the formation of these dark-CPDs was recently elucidated via a three-stage process:[35:1]
- Step 1 involves UV activation of enzymes NADPH oxidase and iNOS to continue to generate nitric oxide (NO•) and superoxide (O2•–) even after UV exposure. These ROS serve as precursors that react at the diffusion-controlled limit to form ONOO-
- Step 2, peroxynitrite then degrades melanin into melanin fragments. ONOO-, melanin and the fragments diffuse into the nucleus and chemically react with each other to form dioxetanes, which are high energy intermediates that are known to degrade thermally to produce excited triplet state carbonyls
- Step 3, these excited triplet state carbonyls then either generate visible luminescence, react with sorbate to generate heat, or transfer energy to nearby DNA bases in the nucleus. This energy transfer generates the same type of excited state as when DNA absorbs UV and subsequently undergo photochemical transformation to form dark-CPDs
The effect of AZ on inhibiting dark-CPDs after UV exposure was measured in melanocytes. The results show that AZ reduced the formation of CPDs within the first two hours post-irradiation.
Six hours post-irradiation results show that levels for both AZ and control were the same, indicating that AZ does not interfere with the cells’ intrinsic DNA repair mechanisms.[1:7]
To further elucidate the mechanism of AZ’s reduction of CPD formation in melanocytes after UV irradiation, Chaudhuri et al. proposed the following target mechanisms:
- Since AZ shows excellent peroxynitrite scavenging ability, it can prevent Stage 1 from progressing to Stage 2.
- In Stage 2, AZ can reduce (donate hydrogen atoms) the dioxetane intermediates to vicinal diols, which was previously noted for a-tocopherol.[38]
- In Stage 3, to monitor the formation of excited triplet state compounds, sodium 9,10-dibromoanthracene-2-sulfonate (DBAS) was used as a triplet state energy acceptor that emits fluorescence. It was also noted that DBAS reduced CPD production by 50-90%.[35:2] For a compound to act as a triplet quencher, the triplet energy should be greater or equal to the triplet state compound’s energy. DBAS monitors energy states greater than 70 – 74 kcal/mol[39], and AZ has triplet energy of 82 kcal/mol[35:3], which is close to that of DBAS. Therefore it is possible that AZ can act as a triplet quencher for Stage 3. Although conceivable, this requires further investigation.
- Another possible way that AZ prevents CPD formation is through quenching of 1O2, as it is known to react with various moieties to form dioxetane intermediates (or other endoperoxides).[40][41][42][43] It was previously thought that 1O2 only exists during irradiation as it only has a short lifespan. However, it was recently reported that endoperoxides could carry 1O2, effectively extending their lifetime. Upon thermal degradation of these carriers in biological systems, they release 1O2, forming more dioxetane intermediates.[44][45] Since AZ has a potent ability to trap 1O2, it can further prevent the formation of dioxetane, which further prevents dark-CPD formation.
Through aging, Advanced Glycation Endproducts (AGEs) accumulate on collagen and elastin, absorbing UV to form excited states. These photoexcited states of proteins modified by AGEs are among the primary endogenous chromophores in the skin (discussed above). In addition to previously discussed mechanisms, AZ physically quenches these excited AGEs by up to 85%, preventing them from forming ROS upon UV exposure.
Anti-aging
AZ is found to affect Extracellular Matrix (ECM) homeostasis, promoting the reactivation of de novo synthesis of matrisome components. Matrisome is the collection of proteins and other structures that promote, regulate, and compose your EMC. They are responsible for keeping your skin smooth and wrinkle-free. AZ works as an anti-aging active through:[2:3]
- Increasing matrisome ( Collagens, Proteoglycans, and ECM) to provide youthful skin
- Decreasing Matrix Metalloproteases (MMPs), which degrades collagen
- Down-regulating Fibroblast Senescence to reactivate collagen production and tone-down skin pigmentation
- Inhibiting pro-inflammatory Biomarkers, which impact collagen production, ECM integrity, and skin pigmentation
Boosting Matrisome Synthesis
ECM is composed of:
Core Matrisome: |
Collagens |
Proteoglycans |
|
ECM Glycoproteins |
|
Proteins Associated with ECM: |
Secreted Factors |
ECM Regulators |
|
ECM Affiliated Proteins |
AZ increased all six of these Matrisome categories, notably increasing collagen-encoding genes by up to 84%. AZ stimulates Collagen 1,3,4 without a concurrent stimulation in metabolic activity and cell proliferation. These increases in mRNA expression correlate with the in-vitro and ex-vivo assays showing corresponding increases in ECM protein abundance. Human dermal fibroblasts were incubated with AZ, and results show that AZ increased protein abundance per unit cell density in a dose-dependent manner (p=<0.05) for:[2:4]
- Collagens (type I, type IC, type VI)
- Fibromodulin (proteoglycan)
- TGF-B (secreted factor)
- Fibronectin (ECM glycoprotein)
- TIMP1 (ECM regulator)
- Vimentin (ECM affiliated)
In the same study, an ex-vivo assay was performed using collagen stains in skin biopsy explants following four days of AZ treatment. Trichrome stain quantification demonstrated 2-fold greater dermal collagen in AZ-treated biopsies.
ECM genes upregulated by AZ:
COL |
Collagen fibers are significant elements of the dermis |
ELN |
Elastin is a self-assembling ECM protein that is the major provider of tissue elasticity |
FBLN1 |
Fibrulin 1 is a calcium-binding EC glycoprotein associated with basement membranes and elastic fibers. It contributes to the elastic properties of connective tissue fibers |
VMN |
Vimentin is required for cell motility, chemotactic migration, and wound healing |
TNS2 |
Tensin 2 regulates cell motility and proliferation |
VCAN |
Versican - key molecule for viscoelasticity of the dermis |
FMOD |
Fibromodulin participates in the assembly of the collagen fibers of the extracellular matrix. FMOD knockout have very fragile skin |
LAM |
Laminins are a major component of the basal lamina, the foundation for most cells and organs, an active part of the basal lamina, and influence cell differentiation, migration, and adhesion |
ITG |
Integrin and ECM expression not only provide epidermal stem cell markers but also regulate stem cell fate. Integrins do regulate epidermal stem cells in vivo |
TIMP |
TIMPs are key regulators of MMPS that degrade ECM and shed cell surface molecules. They are also imp regulators of ECM turnover, tissue remodeling, and cellular behavior |
POT |
Protection of Telomeres - it protects the ends of eukaryotic chromosomes from being recognized as DNA damage and from genetic instability |
MSH |
MutS Homolog 6 - key mismatch repair protein involved in repairing DNA rep errors |
Reduction of MMP activity
As we age, the collagen-rich ECM accumulates damage, which is the hallmark of skin aging. Collagen content is partly determined by the balance between de novo collagen synthesis and breakdown of collagen by MMPs. AZ decreased the expression of MMP1 and MMP3 using RT-PCR. When compared to Zingerone (Z), AZ inhibited MMP3, and MMP12 expression more than Z. Aside from MMP expression downregulation, AZ is also able to decrease the expression of genes related to ECM disassembly (CTSV, NOXO1). Although it seems that AZ downregulates the MMP-1, MMP-3, and MMP-12, it stimulates MMP-2.[2:5] This is why it is vital to have multiple ingredients that cover each other’s weaknesses.
MMP-1 |
degrades collagens 1,2,3,7,8,10 and gelatin[10:2] |
MMP-2 |
degrades basement membrane collagens and degrades denatured structural collagens[10:3] |
MMP-3 |
degrades fibronectin, laminin, collagens 3,4,9, and 10 and cartilage proteoglycans[46] |
MMP-12 |
degrades elastin, fibronectin collagen 4[10:4] |
Fluorescence signal assays revealed the following IC50 values for AZ vs Z:[1:8]
Zingerone |
Acetyl Zingerone |
|
MMP-1 |
0.705 mg |
1.065 mg |
MMP-3 |
0.995 mg |
0.505 mg |
MMP-12 |
0.295 mg |
0.255 mg |
Another interesting result of the RT-PCR was that AZ increased expression of TIMP2. TIMPs are recognized as endogenous regulators of MMPs, a large family of extracellular enzymes with proteolytic activities that participate in cellular homeostasis, adaptation, and tissue remodeling.[47] A balance between MMPs/TIMPs regulates the extracellular matrix (ECM) turnover, and remodeling during normal development and pathogenesis. A good anti-aging strategy is to reduce the MMP/TIMP ratio by decreasing MMPs and increasing TIMPs expression.
Fibroblasts replicative senescence is a crucial feature of skin aging and is associated with decreased collagen production and impaired wound healing.[48] In fact, melasma involves the accumulation of dermal senescent fibroblasts that, if targeted, can result in a significant decrease in hyperpigmentation.[49]
Cellular senescence entails: Irreversible replicative arrest Apoptosis resistance Frequent acquisition of pro-inflammatory tissue-destructive senescence-associated secretory phenotype (SASP)
AZ was found to be negatively correlated with genes observed during senescence. AZ increased genes were biased toward decreased expression during senescence, whereas AZ decreased genes were biased towards increased expression during senescence.[2:6]
Anti-inflammation
Cytokine IL-17A is known to inhibit collagen production through negative regulation of TGF-B responses. AZ expression responses had a strong negative correlation with IL-17A responses with AZ-increased genes biased towards IL-17A decreased expression, and AZ-decreased genes biased toward IL-17A increased expression. AZ mediated inhibition of IL-17A signaling is also linked to downstream repression of NFkB target genes, namely REL and RELA (p<0.05). These suggest that AZ has anti-inflammatory potential. AZ was also able to upregulate Notch and ERK1/2 signaling (regulates bacterial response) and downregulate TGF-B signaling (inflammation).[2:7]
AZ decreased expression of genes expressed during KC differentiation. This effect may be related to inhibition of AP-1 gene transcription,[50] since AZ down-regulated FOS and FOSB expression, and an AP-1 motif was enriched in regions upstream of AZ-decreased genes.[2:8] AZ elicited more retinoid-like responses,[51][52] inhibiting KC differentiation to potentiate a proliferative KC phenotype.[53]
COX dependent prostaglandin E2 (PGE2) is believed to be one of the mediators of UVR-induced erythema; It is considered a rate-limiting step in the generation of leukotrienes prostaglandins. AZ was able to reduce erythema even in low dose concentrations.[2:9]
Protection from Blue light and Pollution-induced ROS
Newer studies found out that UV accounts for only 50% of the Total skin ROS burden while the other 50% is caused by visible light. Even the most protective sunscreen would only cover half of the damages done by the sun.[54][55] In addition to the damages caused by the sun, particulate matter from urban pollution is linked to hyperpigmentation and wrinkle formation.[56] This is why there is a need to wear antioxidant serum every day or possibly even twice a day. Acetyl Zingerone has been shown to decrease Blue Light and Urban Dust-Induced ROS increase in a dose-dependent manner.[57]
Clinical Trials
There was a significant decrease in average wrinkle severity (P = .019; Mean=−25.7% change), total wrinkle volume (P = .003; Mean=−30.1% change), pigment intensity (P = .021; Mean=−25.6% change), and redness intensity (P = .035; Mean=−20.7% change) in the AZ group at 8 weeks compared with the placebo. No significant itching, burning, or stinging was noted by study participants. There was also no significant difference between both groups in the clinical assessment of scaling, erythema, hypopigmentation, or hyperpigmentation.[3:1]
Does it Penetrate?
There are currently no studies on skin penetration of AZ. Because it is relatively small and lipophilic, it is more than likely to penetrate the skin. Since its action is mostly to scavenge and quench free radicals, its presence on the upper layers alone is beneficial. The pharmacodynamics of Zingerone were previously studied, and it showed excellent tissue distribution and good blood-brain barrier penetrance.[4]
References
-
Chaudhuri, R. K., Meyer, T., Premi, S., & Brash, D. (2020). Acetyl zingerone: An efficacious multifunctional ingredient for continued protection against ongoing DNA damage in melanocytes after sun exposure ends. International Journal of Cosmetic Science, 42(1), 36–45. ↩︎ ↩︎ ↩︎ ↩︎ ↩︎ ↩︎ ↩︎ ↩︎ ↩︎
-
Swindell, W. R., Bojanowski, K., & Chaudhuri, R. K. (2020). A Zingerone Analog, Acetyl Zingerone, Bolsters Matrisome Synthesis, Inhibits Matrix Metallopeptidases, and Represses IL-17A Target Gene Expression. In Journal of Investigative Dermatology (Vol. 140, Issue 3, pp. 602–614.e15). https://doi.org/10.1016/j.jid.2019.07.715 ↩︎ ↩︎ ↩︎ ↩︎ ↩︎ ↩︎ ↩︎ ↩︎ ↩︎ ↩︎
-
Dhaliwal, S., Rybak, I., & Pourang, A. (2020). Randomized Double‐Blind Vehicle Controlled Study of The Effects of Topical Acetyl Zingerone on Photoaging. Journal of Cosmetic. https://onlinelibrary.wiley.com/doi/abs/10.1111/jocd.13464 ↩︎ ↩︎
-
Li, L.-L., Cui, Y., Guo, X.-H., Ma, K., Tian, P., Feng, J., & Wang, J.-M. (2019). Pharmacokinetics and Tissue Distribution of Gingerols and Shogaols from Ginger (Zingiber officinale Rosc.) in Rats by UPLC–Q-Exactive–HRMS. Molecules , 24(3), 512. ↩︎
-
Bedard, K., & Krause, K.-H. (2007). The NOX family of ROS-generating NADPH oxidases: physiology and pathophysiology. Physiological Reviews, 87(1), 245–313. ↩︎
-
Shimizu Y, Sakai M, Umemura Y, Ueda H. Immunohistochemical localization of nitric oxide synthase in normal human skin: expression of endothelial-type and inducible-type nitric oxide synthase in keratinocytes. J Dermatol. 1997;24:80–87. ↩︎
-
Bruch-Gerharz D, Ruzicka T, Kolb-Bachofen V. Nitric oxide in human skin: current status and future prospects. J Invest Dermatol. 1998;110:1–7. ↩︎
-
Abd-El-Aleem SA, Ferguson MW, Appleton I, Kairsingh S, Jude EB, Jones K, McCollum CN, Ireland GW. Expression of nitric oxide synthase isoforms and arginase in normal human skin and chronic venous leg ulcers. J Pathol. 2000;191:434–442. ↩︎
-
Wang R, Ghahary A, Shen YJ, Scott PG, Tredget EE. Human dermal fibroblasts produce nitric oxide and express both constitutive and inducible nitric oxide synthase isoforms. J Invest Dermatol. 1996;106:419–427. ↩︎
-
Chaudhuri, R. K. (2020). Skin enhancing compositions and methods (USPTO Patent No. 10568819). In US Patent (No. 10568819). https://patentimages.storage.googleapis.com/73/43/8d/7d9e097f636b0d/US10568819.pdf ↩︎ ↩︎ ↩︎ ↩︎ ↩︎
-
Chistiakov, D. A., Sobenin, I. A., Revin, V. V., Orekhov, A. N., & Bobryshev, Y. V. (2014). Mitochondrial aging and age-related dysfunction of mitochondria. BioMed research international, 2014, 238463. https://doi.org/10.1155/2014/238463 ↩︎
-
Cline S. D. (2012). Mitochondrial DNA damage and its consequences for mitochondrial gene expression. Biochimica et biophysica acta, 1819(9-10), 979–991. https://doi.org/10.1016/j.bbagrm.2012.06.002 ↩︎
-
Beutner, S., Bloedorn, B., Hoffmann, T., & Martin, H. (2000). [22] Synthetic singlet oxygen quenchers. Methods In Enzymology, 226-241. doi: 10.1016/s0076-6879(00)19024-x ↩︎
-
Noctor, G., & Foyer, C. H. (1998). ASCORBATE AND GLUTATHIONE: Keeping Active Oxygen Under Control. Annual Review of Plant Physiology and Plant Molecular Biology, 49, 249–279. ↩︎
-
Edge, R., McGarvey, D. J., & Truscott, T. G. (1997). The carotenoids as antioxidants—a review. Journal of Photochemistry and Photobiology. B, Biology, 41(3), 189–200. ↩︎
-
Stahl, W., & Sies, H. (2003). Antioxidant activity of carotenoids. Molecular Aspects of Medicine, 24(6), 345–351. ↩︎
-
Triantaphylidès, C., & Havaux, M. (2009). Singlet oxygen in plants: production, detoxification and signaling. Trends in Plant Science, 14(4), 219–228. ↩︎
-
Schweitzer, C., & Schmidt, R. (2003). Physical mechanisms of generation and deactivation of singlet oxygen. Chemical Reviews, 103(5), 1685–1757. ↩︎
-
Meyer, T., Beasley, D., & Hanson, K. (2016). Augmenting Skin Photoprotection Beyond Sunscreens. In S. Q. Wang & H. W. Lim (Eds.), Principles and Practice of Photoprotection (pp. 439–460). Springer International Publishing. ↩︎
-
Radi, R. (2013). Peroxynitrite, a stealthy biological oxidant. The Journal of Biological Chemistry, 288(37), 26464–26472. ↩︎
-
Knudsen, F. S., Penatti, C. A. A., Royer, L. O., Bidart, K. A., Christoff, M., Ouchi, D., & Bechara, E. J. H. (2000). Chemiluminescent Aldehyde and β-Diketone Reactions Promoted by Peroxynitrite. Chemical Research in Toxicology, 13(5), 317–326. ↩︎
-
Buettner, G. R. (1993). The pecking order of free radicals and antioxidants: lipid peroxidation, alpha-tocopherol, and ascorbate. Archives of Biochemistry and Biophysics, 300(2), 535–543. ↩︎
-
Lasch, J., Schönfelder, U., Walke, M., Zellmer, S., & Beckert, D. (1997). Oxidative damage of human skin lipids. In Biochimica et Biophysica Acta (BBA) - Lipids and Lipid Metabolism (Vol. 1349, Issue 2, pp. 171–181). https://doi.org/10.1016/s0005-2760(97)00093-3 ↩︎
-
Gungor, H., Ekici, M., Karayigit, M. O., Turgut, N. H., Kara, H., & Arslanbas, E. (2020). Zingerone ameliorates oxidative stress and inflammation in bleomycin-induced pulmonary fibrosis: modulation of the expression of TGF-β1 and iNOS. In Naunyn-Schmiedeberg’s Archives of Pharmacology (Vol. 393, Issue 9, pp. 1659–1670). https://doi.org/10.1007/s00210-020-01881-7 ↩︎
-
Kuo, P.-C., Cherng, C.-Y., Jeng, J.-F., Damu, A. G., Teng, C.-M., Lee, E.-J., & Wu, T.-S. (2005). Isolation of a Natural Antioxidant, Dehydrozingerone from Zingiber officinale and Synthesis of Its Analogues for Recognition of Effective Antioxidant and Antityrosinase Agents. In Archives of Pharmacal Research (Vol. 28, Issue 5, pp. 518–528). https://doi.org/10.1007/bf02977752 ↩︎
-
Rajakumar, D. V., & Rao, M. N. (1994). Dehydrozingerone and its analogues as inhibitors of nonenzymatic lipid peroxidation. Die Pharmazie, 49(7), 516–519. ↩︎
-
Vaibhav, K., Shrivastava, P., Tabassum, R., Khan, A., Javed, H., Ahmed, M. E., Islam, F., Safhi, M. M., & Islam, F. (2013). Delayed administration of zingerone mitigates the behavioral and histological alteration via repression of oxidative stress and intrinsic programmed cell death in focal transient ischemic rats. In Pharmacology Biochemistry and Behavior (Vol. 113, pp. 53–62). https://doi.org/10.1016/j.pbb.2013.10.008 ↩︎
-
Vinothkumar, R., Vinothkumar, R., Sudha, M., & Nalini, N. (2014). Chemopreventive effect of zingerone against colon carcinogenesis induced by 1,2-dimethylhydrazine in rats. In European Journal of Cancer Prevention (Vol. 23, Issue 5, pp. 361–371). https://doi.org/10.1097/cej.0b013e32836473ac ↩︎
-
Whitmore, S. E., Potten, C. S., Chadwick, C. A., Strickland, P. T., & Morison, W. L. (2001). Effect of photoreactivating light on UV radiation-induced alterations in human skin. Photodermatology, Photoimmunology & Photomedicine, 17(5), 213–217. ↩︎
-
Douki, T. (2016). Sunlight-Induced DNA Damage: Molecular Mechanisms and Photoprotection Strategies. In G. T. Wondrak (Ed.), Skin Stress Response Pathways: Environmental Factors and Molecular Opportunities (pp. 49–77). Springer International Publishing. ↩︎ ↩︎
-
Dong, K. K., Damaghi, N., Picart, S. D., Markova, N. G., Obayashi, K., Okano, Y., Masaki, H., Grether-Beck, S., Krutmann, J., Smiles, K. A., & Yarosh, D. B. (2008). UV-induced DNA damage initiates release of MMP-1 in human skin. In Experimental Dermatology (Vol. 17, Issue 12, pp. 1037–1044). https://doi.org/10.1111/j.1600-0625.2008.00747.x ↩︎
-
Tewari, A., Lahmann, C., Sarkany, R., Bergemann, J., & Young, A. R. (2012). Human erythema and matrix metalloproteinase-1 mRNA induction, in vivo, share an action spectrum which suggests common chromophores. Photochemical & Photobiological Sciences: Official Journal of the European Photochemistry Association and the European Society for Photobiology, 11(1), 216–223. ↩︎
-
Walker, S. L., & Young, A. R. (2007). An action spectrum (290–320 nm) for TNFα protein in human skin in vivo suggests that basal-layer epidermal DNA is the chromophore. Proceedings of the National Academy of Sciences of the United States of America, 104(48), 19051–19054. ↩︎
-
Young, A. R., Chadwick, C. A., Harrison, G. I., Nikaido, O., Ramsden, J., & Potten, C. S. (1998). The similarity of action spectra for thymine dimers in human epidermis and erythema suggests that DNA is the chromophore for erythema. The Journal of Investigative Dermatology, 111(6), 982–988. ↩︎
-
Premi, S., Wallisch, S., Mano, C. M., Weiner, A. B., Bacchiocchi, A., Wakamatsu, K., Bechara, E. J. H., Halaban, R., Douki, T., & Brash, D. E. (2015). Chemiexcitation of melanin derivatives induces DNA photoproducts long after UV exposure. In Science (Vol. 347, Issue 6224, pp. 842–847). https://doi.org/10.1126/science.1256022 ↩︎ ↩︎ ↩︎ ↩︎
-
Delinasios, G. J., Karbaschi, M., Cooke, M. S., & Young, A. R. (2018). Vitamin E inhibits the UVAI induction of “light” and “dark” cyclobutane pyrimidine dimers, and oxidatively generated DNA damage, in keratinocytes. Scientific Reports, 8(1), 423. ↩︎
-
Lawrence, K. P., Douki, T., Sarkany, R. P. E., Acker, S., Herzog, B., & Young, A. R. (2018). The UV/Visible Radiation Boundary Region (385–405 nm) Damages Skin Cells and Induces “dark” Cyclobutane Pyrimidine Dimers in Human Skin in vivo. In Scientific Reports (Vol. 8, Issue 1). https://doi.org/10.1038/s41598-018-30738-6 ↩︎
-
Adam, W., Vargas, F., Epe, B., Schiffmann, D., & Wild, D. (1989). Single-Electron-Transfer in the Reduction of 1, 2-Dioxetanes by Biologically Active Substrates. In Free Radical Research Communications (Vol. 5, Issues 4-5, pp. 253–258). https://doi.org/10.3109/10715768909074708 ↩︎
-
Catalani, L. H., Wilson, T., & Bechara, E. J. H. (1987). TWO WATER-SOLUBLE FLUORESCENCE PROBES FOR CHEMIEXCITATION STUDIES: SODIUM 9,10-DIBROMO- AND 9, 10-DIPHENYLANTHRACENE-2-SULFONATE. SYNTHESIS, PROPERTIES AND APPLICATION TO TRIPLET ACETONE AND TETRAMETHYLDIOXETANE. In Photochemistry and Photobiology (Vol. 45, Issue 2, pp. 273–281). https://doi.org/10.1111/j.1751-1097.1987.tb05375.x ↩︎
-
Foote, C. S., & Darling, T. R. (1975). Decomposition of dioxetanes: a unique probe into mechanism and energy transfer processes. In Pure and Applied Chemistry (Vol. 41, Issue 4, pp. 495–506). https://doi.org/10.1351/pac197541040495 ↩︎
-
Mazur, S., & Foote, C. S. (1970). Chemistry of singlet oxygen. IX. Stable dioxetane from photooxygenation of tetramethoxyethylene. In Journal of the American Chemical Society (Vol. 92, Issue 10, pp. 3225–3226). https://doi.org/10.1021/ja00713a073 ↩︎
-
Saito, I., Matsugo, S., & Matsuura, T. (1979). Photoinduced reactions. 108. 1,2-dioxetane formation in an indole system. In Journal of the American Chemical Society (Vol. 101, Issue 16, pp. 4757–4759). https://doi.org/10.1021/ja00510a068 ↩︎
-
Zhang, X., & Foote, C. S. (2010). ChemInform Abstract: 1,2-Dioxetane Formation in Photooxygenation of N-Acylated Indole Derivatives. In ChemInform (Vol. 25, Issue 7, p. no – no). https://doi.org/10.1002/chin.199407134 ↩︎
-
Pierlot, C., Aubry, J.-M., Briviba, K., Sies, H., & Di Mascio, P. (2000). [1] Naphthalene endoperoxides as generators of singlet oxygen in biological media. In Methods in Enzymology (pp. 3–20). https://doi.org/10.1016/s0076-6879(00)19003-2 ↩︎
- ↩︎
-
Lu, P., Takai, K., Weaver, V. M., & Werb, Z. (2011). Extracellular matrix degradation and remodeling in development and disease. Cold Spring Harbor perspectives in biology, 3(12), a005058. https://doi.org/10.1101/cshperspect.a005058 ↩︎
-
Moore, C. S., & Crocker, S. J. (2012). An alternate perspective on the roles of TIMPs and MMPs in pathology. The American Journal of Pathology, 180(1), 12–16. ↩︎
-
Tigges, J., Krutmann, J., Fritsche, E., Haendeler, J., Schaal, H., Fischer, J. W., Kalfalah, F., Reinke, H., Reifenberger, G., Stühler, K., Ventura, N., Gundermann, S., Boukamp, P., & Boege, F. (2014). The hallmarks of fibroblast ageing. Mechanisms of Ageing and Development, 138, 26–44. ↩︎
-
Yoon, J. E., Kim, Y., Kwon, S., Kim, M., Kim, Y. H., Kim, J.-H., Park, T. J., & Kang, H. Y. (2018). Senescent fibroblasts drive ageing pigmentation: A potential therapeutic target for senile lentigo. Theranostics, 8(17), 4620–4632 ↩︎
-
Wurm, S., Zhang, J., Guinea-Viniegra, J., García, F., Muñoz, J., Bakiri, L., Ezhkova, E., & Wagner, E. F. (2015). Terminal epidermal differentiation is regulated by the interaction of Fra-2/AP-1 with Ezh2 and ERK1/2. Genes & development, 29(2), 144–156. https://doi.org/10.1101/gad.249748.114 ↩︎
-
Kang, S., Duell, E. A., Fisher, G. J., Datta, S. C., Wang, Z. Q., Reddy, A. P., Tavakkol, A., Yi, J. Y., Griffiths, C. E., & Elder, J. T. (1995). Application of retinol to human skin in vivo induces epidermal hyperplasia and cellular retinoid binding proteins characteristic of retinoic acid but without measurable retinoic acid levels or irritation. The Journal of investigative dermatology, 105(4), 549–556. https://doi.org/10.1111/1523-1747.ep12323445 ↩︎
-
Shao, Y., He, T., Fisher, G. J., Voorhees, J. J., & Quan, T. (2017). Molecular basis of retinol anti-ageing properties in naturally aged human skin in vivo. International journal of cosmetic science, 39(1), 56–65. https://doi.org/10.1111/ics.12348 ↩︎
-
Eichner R. (1986). Epidermal effects of retinoids: in vitro studies. Journal of the American Academy of Dermatology, 15(4 Pt 2), 789–797. https://doi.org/10.1016/s0190-9622(86)70235-1 ↩︎
-
Zastrow, F.L., Groth, N., Klein, F., Kockott, D., Lademann, J., Renneberg, R. and Ferraro, L. (2009). The missing link – light induced (280 nm–1600 nm) free radical formation in human skin. Skin Pharmacol Physiol 22 31-44. ↩︎
-
Liebel, F., Kaur, S., Ruvolo, E., Kollias, N. and Southall, M.D. (2012). Irradiation of skin with visible light induces reactive oxygen species and matrix-degrading enzymes. J Invest Dermatol 132 1901-1907. ↩︎
-
Vierkotter, A., Schikowski, T., Rannft, U., Sugiri, D., Matsui, M., Kramer, U. and Krutmann, J. (2010). Airborne particle exposure and extrinsic skin aging. J Invest Dermatol 130 (12) 2719-2726. ↩︎
-
Meyer, T., Chaudhuri, R. (2020). Acetyl Zingerone Scavenges/Quenches Reactive Species, Selectively Chelates Iron. Cosmetics and Toiletries. 135;9 24-36. ↩︎